Ambient noise data collection
The main objective of WP1 is the collection of land-based noise records and aims to create a model dataset for the two WPs implementing the 2 main project objectives. In particular:
Α) The data collection for the implementation of 3D ground noise tomography will be carried out by the temporary installation of dedicated seismometer networks in two sites, first in free field conditions outside the city and then in an urban environment. The first application (free-field conditions) will be carried out in the Mygdonia basin (Euroseistest test site, http://euroseisdb.civil.auth.gr/), following previous research in the area (Hannemann et al. 2014), but using a different sensor configuration to optimise the coverage area and wavelength range. The application in an urban environment concerns the central part of the Thessaloniki urban complex, for which encouraging results are already available (Anthymidis et al, 2018), but also available geophysical/geotechnical results from previous large-scale studies (Anastasiadis et al. 2001, Apostolidis et al. 2004, Panou et al. 2005). For the acquisition of the measurements it was planned to use the WARAN infrastructure of the University of Potsdam, (http://seismo.geo.uni-potsdam. de/waranproj), which allows the collection, transmission and automatic analysis of seismic recordings in the field in real time, and is therefore suitable for taking ground noise measurements in accordance with the agreement signed between the partner institution and the member of the research team (Dr. Matthias Ohrnberger, Institut für Geowissenschaften, University of Potsdam). This infrastructure could not be used because it was not possible to conclude the relevant service contract between Arist. University of Thessaloniki (AUTH) and the University of Potsdam (UoP), without this being the fault of the two institutions. In particular, under current Greek legislation, the relevant service contract of the UoP to the AUTH should have been drawn up in Greek, or in the case of a bilingual contract (e.g. Greek and English), the predominant language should be Greek. This provision was not accepted by the legal department of the University of Potsdam, which reasonably requested an international language (e.g. English) as the sole and dominant language. Despite extensive correspondence, no solution could be found to the above problem, which arises from the existing Greek legislation, resulting in the non-use of the equipment in question, causing additional delay and damage to the project.
B) The ground noise measurements to be used for 1D inversion will be carried out at selected locations in the Greek area, from the Stewart et al. (2014) one-dimensional reference model database, where a large amount of geophysical/geotechnical information is available. This database has already been exploited in a previous study (Chatzis et al., 2018) to calibrate HVSR curve inversions from single station noise measurements. Within the project, measurements will be carried out at 30 selected locations, using a dedicated network of seismometers (arrays), to enable the retrieval of local surface wave dispersion curves.
As can be understood, the project includes a large part of experimental field work, with the collection of a very large number of sample data from seismograph arrays in a significant number of urban and non-urban complexes in the Greek territory (WP1). The conditions created for more than 50% of the project time due to the emergency measures to protect public health from the risk of further spreading of the COVID-19 coronavirus, did not allow to keep to the project execution schedule. In particular, for a long time there was a complete travel ban, which practically prevented any measurement from being carried out. Moreover, even when travel was conditionally allowed, it was impossible to move the whole staff for measurements using the same means of transport, due to the existing restrictions. At the same time, part of the staff either became ill from COVID-19, or were very reluctant or even reasonably reluctant (e.g. due to the presence of persons from vulnerable groups in their homes) to participate in experiments that required long stays in cars to travel to the measurement sites and other locations (e.g. closed installation sites in urban areas), even when travel was allowed. The same was true for travel abroad (partner organisation), which was very late due to the relevant prohibitions.
The restrictions that arose in carrying out the field measurements caused very great problems in their implementation, even when this was possible. In particular, the WP1 measurements with ambient noise measurement networks require the simultaneous presence of 5-8 people in the field (for standard ambient noise network measurements) and 20-25 people for tomographic measurements in urban/peri-urban environments, to ensure the operation and safety of the equipment, which must remain and record from 8 to 48 (or more) hours. For this reason, both the proposal and the approved project TOR had foreseen the participation of additional staff, outside the Research Team.
In particular, the ToR had provided and approved that: “Additional permanent staff of the Geophysics Laboratory of the Aristotle University of Thessaloniki, as well as undergraduate and postgraduate students will participate in the project, exclusively to support/assist in the data collection process. No personnel costs are foreseen for these staff, as they will not be members of the research team and their involvement concerns project support activities, for which only travel costs will be covered (mobility, use of car and fuel, accommodation, daily living expenses, etc.).”
Despite the approval of the ToR, the above provision was not implemented, as it was not allowed by the Financing Guide, which foresees the coverage of expenses only for the needs of project team members. It is obvious that this need could not be met by recruiting a very large number of additional members to the project team, since tomographic measurements require a very large number of collaborators and this cannot be arranged in advance (e.g. by contracting) due to the limitations of such an effort to coordinate a very large number of people (e.g. often several members of the Geophysics Project Team or students were sick with COVID-19, had other inelastic commitments, etc.). Therefore, the implementation of the WP1 measurements (WP1) became extremely difficult within the project.
As mentioned, the objectives of WP1 are: a) the design of the dedicated seismometer array for the acquisition and recording of ground noise data to be used to create 3D models for WP2 and, b) the collection of ground noise data at calibration sites for use in the one-dimensional geophysical inversion models of WP3.
In the context of these objectives, during the first phase of the project the following were carried out:
α) The design of the possible geometry-layout of temporary special seismometer arrays, both in free field conditions outside the city (Mugdonia basin) and in urban environment (central part of the Thessaloniki urban complex) for the application of 3D ground noise tomography. The seismometer array was based on available geological, geophysical and geotechnical information, as well as on previous large-scale work in the study area (e.g. Apostolidis et al. 2004, Panou et al. 2005, Skarlatoudis et al. 2010). Also, the design and spatial distribution of seismometers (optimization of station geometry) was carried out considering already existing information on the local structure using synthetic data.
According to this information, and for the layout concerning the urban complex of Thessaloniki (application in an urban environment), the geological formations follow a generally two-dimensional (2D) development, where the rocks of the geological background (mainly greenschist) from their surface occurrence in the NE of the study area, sink towards the SW and are covered by thick modern sedimentary deposits. This sedimentary cover in the most SW parts (near the city’s waterfront) is likely to be more than 200m deep. As part of the project and to effectively cover the study area and produce tomographic images and geophysical models of the subsurface to illustrate the specific lateral transition of the geological basement, a total of thirty (30) ground noise recording stations were installed at suitably selected locations. These locations extend both to areas of surface outcrops of the geologic basement and to areas where large thicknesses of sedimentary deposits are expected. The stations used were provided by the Implementing Agency and a number of other Greek institutions (ITSSAK-OASP, Erg. Erdrodynamics Aristotle University of Thessaloniki, etc.), since it was not possible to use the WARAN infrastructure of the University of Potsdam.
Figure 1.1 shows the geometry of the installed dedicated seismometer network in the urban complex of Thessaloniki, which was used for the collection of ground noise recordings in the framework of WP1. The minimum distance between the stations was approximately 100m, while the maximum distance was approximately 1400m. In addition, the geometry of the specific network allowed for a sufficient density of paths or rays covering the study area, as well as an adequate azimuthal distribution. These two parameters are necessary conditions for the reliable application of the subsurface tomography in the next section of work (WP2). Figure 1.2 shows the ray coverage resulting from the geometry of the installed specific network in the study area, while Figure 1.3 and Figure 1.4 show the histograms of the distances between the ground noise recording stations and the azimuth of the rays, respectively.
The dedicated network stations installed to collect ground noise data consisted of an integrated system of seismological instruments that provided the capability to record and store ground motion. In addition, these systems had the appropriate support for the use of the Global Positioning System (GPS) to enable the geographic coordinates of the stations to be found with high accuracy, as well as the synchronisation of each station with Coordinated Universal Time (UTC). More specifically, three-component, high-resolution and broadband seismometers were used. In total, three different combinations of sensors and digitizers were used. The first combination consisted of the Güralp CMG-40T sensor coupled with the Reftek 130-01 digitizer, while the second consisted of the Güralp CMG-6TD integrated sensor-digitizer system. Finally, the third combination used Nanometrics’ Trillium Compact 120 sensors together with Centaur digitizers. It is noted that all sensors were velocimeters, with 24-bit resolution digitizers.
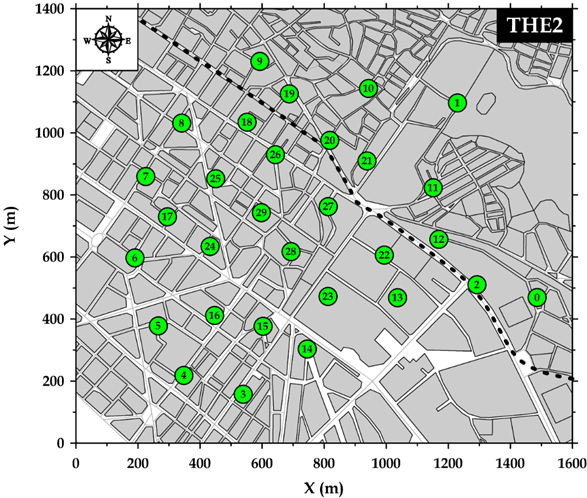
Figure 1.1: Installed dedicated seismometer network for ground noise recording in the study area under WP1. The locations of the ground noise recording stations are shown in green circles. Each station in the dedicated network is marked with its identification number. The black dotted line corresponds to the surface trace of the contact of the geological basement (greenschist, towards the north-east) with recent sedimentary formations.
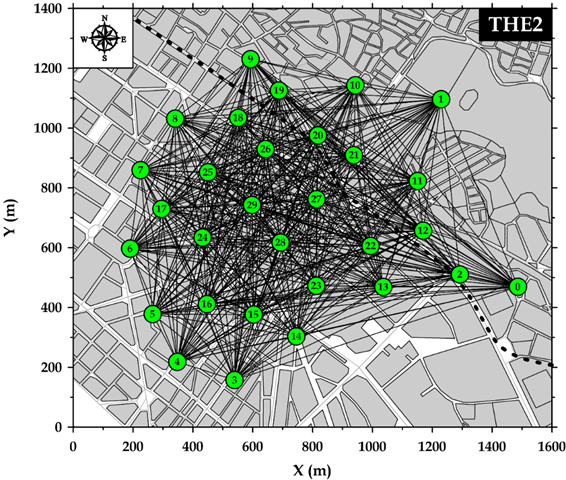
Figure 1.2: Coverage of paths or rays, as derived from the geometry of the specific seismometer network in the study area. The ray density throughout the spatial extent of the network is high, with the exception of the peripheral part of the network. The locations of the ground noise recording stations are shown in green circles. Each station in the specific network is marked with its characteristic identification number. The black dotted line corresponds to the surface trace of the contact between the geological background (greenschist, towards the north-east) and recent sedimentary formations.
The installation of the dedicated network stations was mainly carried out inside buildings to protect them, to avoid the influence of severe weather events on the recordings, and to avoid anthropogenic interference. The recording of ground noise at each station was continuous, with a sampling rate set at 100 Hz. The period of recordings ranged from a few days to three (3) months, with the majority of the stations in the dedicated network having data for more than thirty (30) days.
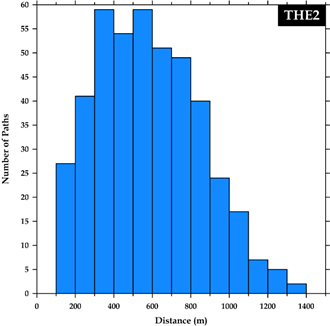
Figure 1.3: Histogram of distances between the ground noise recording stations of the installed dedicated seismometer network in the study area.
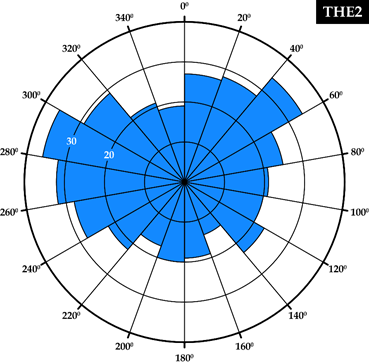
Figure 1.4: Azimuthal distribution of the rays connecting each pair of ground noise recording stations in the installed dedicated seismometer network in the study area.
b) The selection of 30 reference sites for ground noise measurements of a dedicated network for use for 1D inversion considering the different geological-geotechnical-geophysical conditions and the available information of each site from the database of Stewart et al. (2014). During the reference period, a total of 11 ground noise measurements were performed using a dedicated seismometer network (noise Array). Table 1.1 shows the geographic coordinates of the locations with their respective names. Also given are the reference method from which the known structure models are derived expressed in terms of transverse wave velocities, the maximum depth of the available reference structure models, the natural frequency (f0) of the ground as derived from the noise measurements, the depth of the background for those cases where this was known, and finally the average transverse wave velocity from the surface to the maximum depth of the reference structure model. It is worth noting that locations lacking a reference structure model will be calibrated with independent geological data. A typical example is the MEG site, for which geological information (geological stratigraphy) is available from the caldera slopes in Santorini.
Table 1.1: Locations of the 11 ground noise measurements from a dedicated seismometer network (noise Array). From left to right are given the geographical coordinates of the locations, the corresponding code names, the method used to calculate the reference structure model of the transverse waves, the maximum depth of the reference structure, the natural frequency of each location (from the project measurements), the depth of the background (from the reference model) and the average velocity of the transverse waves from the surface to the maximum depth of the structure model.
Latitude | Longitude | Site code | Method | Max. depth | f0 (Hz) | Bedrock depth(m) | VSZ |
40.63173 | 22.9628 | THETHE013b | Downhole | 22 | 20 | 0 | 742 |
41.07078 | 24.763833 | NESXAN001a | Crosshole | 64 | 1.4 | 52 | 364 |
41.0705 | 24.756333 | NESXAN005a | Crosshole | 38 | 1.8 | 35 | 256 |
41.16675 | 22.900493 | KNTXER001e | MASW | 35 | 20 | 0 | 743 |
40.30493 | 21.783974 | KZNKZN003b | Downhole | 38 | 3.5 | – | 543 |
40.29324 | 21.783444 | KZNKZN013b | Downhole | 42 | 1.2 | 80-100 | 454 |
36.34884 | 25.453944 | EFR | – | – | 1 | – | – |
36.37846 | 25.432988 | MEG | Caldera Cliff | – | 1.26 | 130 | – |
36.36109 | 25.43067 | SXL | – | – | 1.3 | – | – |
36.39653 | 25.461313 | KTS | – | – | 1.15 | – | – |
36.42031 | 25.434654 | FIR | – | – | 0.34 | – | – |
Figures 1.5 and 1.6 show the spatial distribution of noise measurements from specific seismometer networks. In particular, Figure 1.5 shows the locations of the measurements in the northern Greek area, while Figure 1.6 shows the locations on the island of Santorini. The main features of the geology and surface geophysical structure for which these locations were selected are as follows:
-
- KNTXER001e and THETHE013b are instances of transformed background. In particular, the first site consists of Palaeozoic-age bimargal gneisses of the Servomacedonian Mass, while the second site consists of the Mesozoic epigneisses of the Thessalonica, part of the Peridotopian belt.
-
- Sites NESXAN001a and NESXAN005a consist of alluvial Holocene sediments over metamorphic gneisses, which are part of the Rhodope Massif.
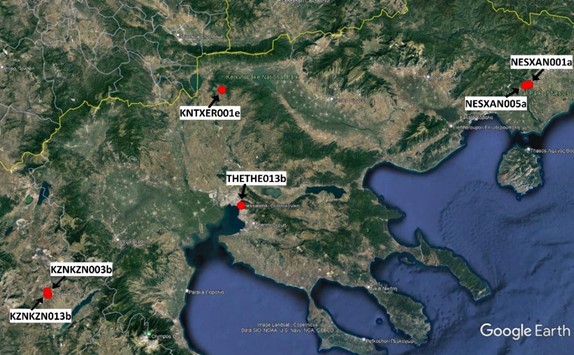
Figure 1.5. Map of northern Greece with the locations of ground noise measurements from a special network of seismometers.
-
- Sites KNZKZN003b and KZNKZN013b in the city of Kozani consist of sedimentary Pliocene rocks, which overlie felsic and carbonate limestone rocks of middle-to-upper Cretaceous age of the Pelagonian zone.
Sites FIR, KTS, MEG, SXL and EFR consist mainly of pyroclastic deposits of the first and second eruptive cycle of the Santorini volcano, aged 3.6-360ka. The upper part of the pyroclastic deposits consists of the Minoan tops, characteristic of the Minoan eruption, which occurred at the end of the Bronze Age (~1560 BC, according to the most recent excavations). The pyroclastic deposits overlie the metamorphic basement of the Attic-Cycladic zone, consisting of shales and recrystallized limestones, aged in the Palaeocene-Eocene and Upper Triassic, respectively.
The 11 locations of ground noise measurements from a dedicated network of seismometers were carried out using a wide range of Guralp-6TD seismometers/seismographs. These seismometers provide the recording of ground motion in three components, one vertical and two horizontal (north-south, east-west) with a constant (flat) spectral response from 0.05 to 100Hz. It is worth noting that, the seismometers were tested for the reliability of their response before the start and during the measurements, by taking noise recordings from them in a common position (Huddle Test). Figure 1.7 shows the spectral response of the vertical (Z) components of the Guralp-6TD seismometers from common position noise recordings.
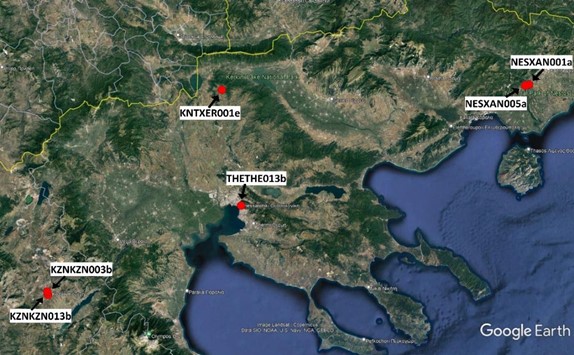
Figure 1.6. Map of the Santorini volcano with the locations of ground noise measurements from a special seismometer array.
As can be seen from this figure, the seismometers show similar spectral response for the frequency range from 0.2 to 20 Hz. However, a significant deviation is observed in the curve of seismometer 6695 from the other curves, due to the fact that the response of this seismometer was set to short period instead of wide range. Similar problems were identified by the Huddle Test on all measurements and were addressed-corrected prior to taking measurements at each location.
Noise measurements from a dedicated network of seismometers are made by simultaneously recording noise from a number (usually 6) of seismometers placed around a central seismometer. Both the geometry of the seismometer layout and the maximum and minimum distance of the seismometers from the central station determine the so-called theoretical response of the network, i.e. the maximum and minimum depth of the survey. The design of the measurements of each location was made taking into account the available structure model (reference model) of the transverse wave velocities, from the database of Stewart et al. (2014), but also inversion results of the Rayleigh wave ellipticity and the ground natural frequency (f0) from the database of Chatzis et al. (2018). Figure 1.8 shows a typical example of a known geophysical structure with the variation of seismic (transverse and longitudinal) wave velocities with depth for NESXAN001a. The theoretical scattering curve resulting from the structure of NESNESXA001a is given in Figure 1.9. Also given in this figure is the
Figure 1.7: Spectral response curves of the vertical components of the Guralp-6TD seismometers.
maximum (continuous curve) and minimum (dashed curve) reliability limits of the theoretical network response, which is derived from the geometry chosen for this location, in order to ensure that the theoretical scattering curve (and its expected main variations) are within these limits of resolution of the specific setup.
Figure 1.10 shows the geometry of the seismometer array used for the noise measurements from a dedicated seismometer network for NESXAN001a. In this, three seismometers (triangular array) were placed at distances of 20, 60 and 200m from the main seismometer in order to simultaneously record ambient noise. Detailed information on the geometries of the arrays used in the measurements of each site are included in Table 1.2. Three deployments were carried out in the measurements of the northern Greek sites, while four deployments were carried out in the measurements on the island of Santorini. The exception is the FIR site, where noise measurements were made using two large deployments (200 and 500m), as there were measurements of a specific seismometer network from previous experiments with shorter distance deployments.
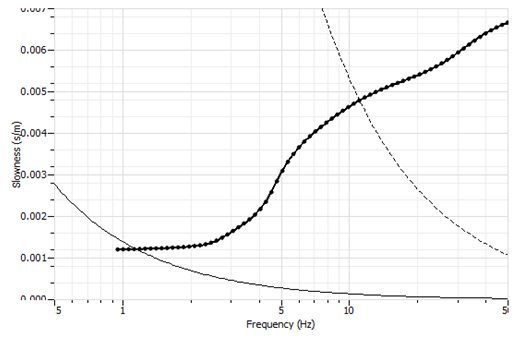
Figure 1.9 Theoretical scattering curve for NESXAN001a, as derived from the seismic wave velocity structure in Figure 1.8. The maximum and minimum confidence limits of the theoretical network response based on the measurement geometry chosen for this location (Figure 1.10, Table 1.2) are given by a solid and dashed black curve, respectively.
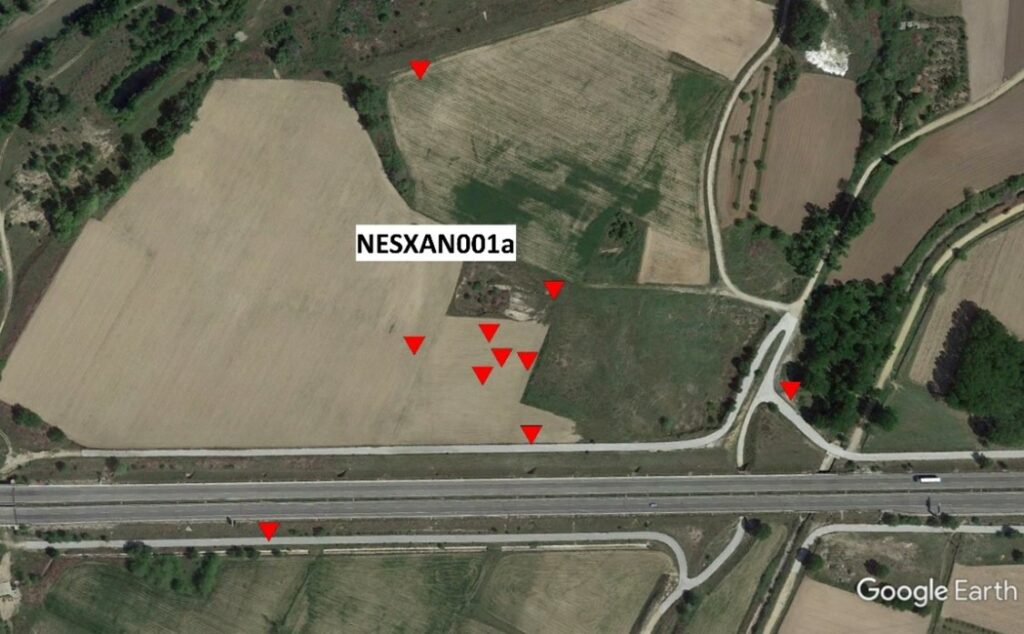
Figure 1.10: Geometry of the seismometer layout used in the ground noise measurements from a dedicated seismometer network at NESXAN001a. The geometry chosen is three triangular deployments. Each deployment includes three seismometers, which are placed at a distance of 20, 60 and 200 m from the central seismometer.
Table 1.2: Final geometries used in the ground noise measurements from a dedicated seismometer network. The number of seismometers used in each deployment is given, as well as their distance from the central seismometer.
Site code | Aperture1 | Aperture2 | Aperture3 | Aperture4 | ||||
Sensors | Radius(m) | Sensors | Radius(m) | Sensors | Radius(m) | Sensors | Radius(m) | |
THETHE013b | 6 | 10 | 3 | 30 | 3 | 100 | – | – |
NESXAN001a | 3 | 20 | 3 | 60 | 3 | 200 | – | – |
NESXAN005a | 3 | 20 | 3 | 60 | 3 | 200 | – | – |
KNTXER001e | 3 | 20 | 3 | 60 | 3 | 200 | – | – |
KZNKZN003b | 3 | 15 | 3 | 50 | 3 | 200 | – | – |
KZNKZN013b | 3 | 15 | 3 | 50 | 3 | 200 | – | – |
EFR | 6 | 15 | 3 | 50 | 3 | 150 | 3 | 400 |
MEG | 3 | 15 | 3 | 50 | 3 | 150 | 3 | 400 |
SXL | 6 | 15 | 3 | 50 | 3 | 150 | 3 | 400 |
KTS | 3 | 15 | 3 | 50 | 3 | 150 | 3 | 400 |
FIR | – | – | – | – | 3 | 200 | 3 | 500 |
In summary, as regards the actions (Tasks) mentioned in the approved TOR for WP1, the following have been implemented:
-
- Design and optimization of the geometry of the 3D layout of dedicated seismometer networks, taking into account the available a priori information on the local structure, using synthetic data
Both specific networks (urban environment, city of Thessaloniki) and (peri-urban environment, Mygdonia basin) have been fully implemented.
-
- Selection of reference sites to perform 1D measurements of specific noise networks, sampling/selection of different geological/geotechnical/geophysical conditions
It has been fully implemented after a full data analysis of the results of Stewart et al. (2014) and Chatzis et al. (2018), and all sites have been selected.
-
- Transfer of WARAN equipment from UoP to Aristotle University. Thessaloniki, equipment testing and staff training
The transfer of the WARAN infrastructure of the University of Potsdam did not take place, (http://seismo.geo.uni-potsdam.de/waranproj) at the Aristotle University of Thessaloniki, for reasons explained in paragraph A.8.This resulted in the delay of the installation of the ground noise recording networks (3D and 1D) and the finding of an alternative solution (by other operators) for the realization of the measurements implemented so far.
-
- Implementation of two (2) 3D measurements of specific noise networks in the Mygdonia basin and in the city centre of Thessaloniki, to provide data for WP2
As mentioned above, one specific network (urban environment, city of Thessaloniki) has been implemented and the measurements for the second specific network (peri-urban environment, Mygdonia basin) have been carried out. In particular, the networks were installed in the urban environment in the central part of the urban complex of Thessaloniki and in the peri-urban environment of the Mygdonia basin (Euroseistest test area),(http://euroseisdb.civil.auth.gr/) using ground motion data loggers of the Geophysics Laboratory of the Department of Geology of the Aristotle University of Thessaloniki, the Laboratory of Soil Mechanics, Foundations and Geotechnical Seismic Engineering of the Department of Civil Engineering of the Aristotle University of Thessaloniki and the Institute of Technical Seismology and Earthquake Engineering (OASP/ITSSAK).
-
- Perform ambient noise network measurements to determine 1D Vs patterns at the selected calibration sites for WP3
11 measurements have been implemented in the first 18 months (reporting time of this report) due to COVID-19 limitations and inability to use the WARAN device. At the time of writing this report 4 more measurements had been implemented and the last 14 measurements have been initiated.
-
- Carry out additional/alternative noise measurements, based on the preliminary data pre-processing results from WP2 and WP3
Additional measurements will depend on the results of WP2 and WP3. At this stage, the need for additional measurements is not apparent, but this cannot be excluded in the future.
Given that the planned employment of staff to carry out the WP1 measurements (WP1), which is necessary to complete, did not materialise, there should be additional members in the working group, which will be used within WP1 to complete the collection of measurements. In addition to the planned deliverables, the project is expected to complete additional deliverables, which have emerged as new opportunities in the context of the research that has been carried out and which further the objectives of the project. With regard to WP1, the new deliverable will be P1.2 (D1.2), which will be the additional calibration/comparison of WP1 measurements with national databases.
Anastasiadis A, Raptakis D, Pitilakis K (2001). Thessaloniki’s detailed microzoning: subsurface structure as basis for site response analysis, Pure Appl. Geophys., 158,2597–2633, doi: 10.1007/PL00001188
Apostolidis P, Raptakis D, Roumelioti Z, Pitilakis K (2004). Determination of S-wave velocity structure using microtremors and SPAC method applied in Thessaloniki (Greece), Soil Dyn. Earthq. Eng., 24,49–67, doi: 10.1016/j.soildyn.2003.09.001.
Chatzis, N., Papazachos, C., Theodoulidis, N., Klimis, N., and Anthymidis, M. (2018) Determination of 1D Velocity Models by Single-Station Ambient Noise Data Inversion and Joint Interpretation with Independent Geological, Geotechnical and Geophysical Information. 16th Eur. Conf. Earth. Eng., Thessaloniki.
Hannemann, K., Papazachos, C., Ohrnberger, M., Savvaidis, A., Anthymidis, M. and Lontsi, A.M.,2014. Three-dimensional shallow structure from high-frequency ambient noise tomography:New results for the Mygdonia basin-Euroseistest area, northern Greece, J. Geophys. Res. Solid.Earth, 119, doi: 10.1002/2013JB010914.
Panou, A.A., Theodulidis, N., Hatzidimitriou, P., Stylianidis, K. and Papazachos, C.B., 2005. Ambient noise horizontal-to-vertical spectral ratio in site effects estimation and correlation with seismic damage distribution in urban environment: the case of the city of Thessaloniki (Northern Greece). Soil Dynamics and Earthquake Engineering, 25(4), 261-274.
Skarlatoudis, A.A., Papazachos, C.B., Theodoulidis, N., Kristek, J. and Moczo, P., 2010. Local site-effects for the city of Thessaloniki (N. Greece) using a 3-D finite-difference method: a case of complex dependence on source and model parameters. Geophysical Journal International, 182(1), pp.279-298.
Stewart J-P, Klimis N, Savvaidis A, Theodoulidis N, Zargli E, Athanasopoulos G, Pelekis P, Mylonakis G, Margaris B (2014). Compilation of a Local VS Profile Database and Its Application for Inference of VS30 from Geologic- and Terrain-Based Proxies. Bulletin of the Seismological Society of America, 104(6), 2827-841.